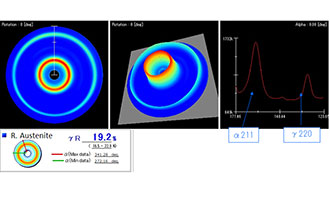
In manufacturing, it’s common to hear steels described as either martensitic or austenitic. These are not just scientific terms but rather key components in understanding the behavior of steel itself.
This article will describe the primary differences between martensite and austenite, including their primary characteristics, how they’re formed, and how they each impact the mechanical properties of steel.
Table of Contents
What Is Martensite?
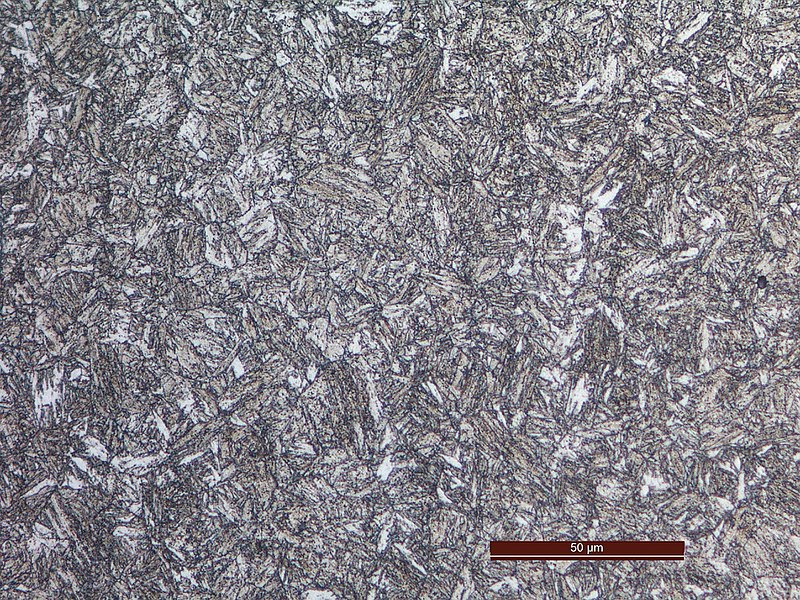
Martensite is an extremely hard yet brittle form of steel with a body-centered tetragonal (BCT) crystal structure.
Even with its brittle nature, martensite is not inherently “bad.” While its brittleness can be a disadvantage in some applications, it’s not necessarily a drawback in all scenarios. Martensitic steel is hard with a resistance to slip, making it ideal in specific applications requiring rigidity and strength.
Additionally, martensitic stainless steel contains between 11.5 to 18% chromium and 1.2% carbon, making it incredibly durable, strong, corrosion-resistant, and a viable choice for many industrial applications.
Causes
The creation of martensite is a matter of temperature manipulation. When austenite—a gamma-phase iron—is rapidly cooled or quenched, it transforms into martensite through a diffusionless phase transformation.
This quick transition leaves no time for carbon atoms to break away from the austenite, resulting in the formation of a martensitic structure that is supersaturated with carbon and rich in dislocations, which is the primary factor behind its strength. It’s important to note that to fully transform austenite into martensite before pearlite (a lamellar or layered structure in steel) can form, a very rapid quench is needed.
The transformation is not just chemical but also physical, where a volume change occurs during the process due to the lower density of martensite compared to austenite. But remember, martensite is not an equilibrium phase. Its structure is easily modified by heat treatments, like tempering, which can be used to reduce brittleness by decreasing martensite content. This becomes an essential control in the hardness of heat-treated steel samples.
Measuring Martensite
One of the most common ways to non-destructively measure martensite in steel is X-ray diffraction. Martensite and other phases in steel have characteristic peak positions and intensities. When measuring martensite via XRD, you would use the peak data to quantify the amount of martensite. This might involve comparing the intensities of specific peaks corresponding to martensite with those of other phases and then interpreting this data in the context of the steel’s treatment and expected microstructure.
Austenite
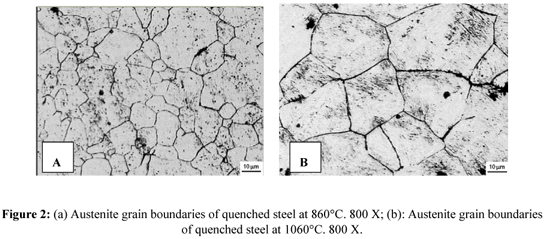
Austenite, also referred to as gamma-phase iron, is a non-magnetic allotrope of iron or a solid solution of iron with alloying elements. Characterized by its face-centered cubic (FCC) crystal structure, austenite exists above the critical eutectoid temperature of 1000 Kelvin (727 degrees Celsius) in plain-carbon steel.
Austenitic steel is a particular class of stainless steel that primarily contains austenite. What sets it apart is the high percentage of nickel and chromium that give it strength and an impeccable resistance to corrosion. A significant percentage of manganese and nitrogen may also be present in austenitic steels, and stainless steels with an 18/8 grade having 18% chromium and 8% nickel are the most common type.
This type of steel is renowned for its high deformability and excellent formability. It can be easily formed and welded into any shape, making it an ideal choice for industrial manufacturing. Due to its high alloy content, it remains austenitic even at room temperature, making austenitic stainless steels non-magnetic when annealed and displaying slight magnetic properties when cold-worked.
Causes
Austenite formation occurs during a process termed “austenitization.” In this process, iron, steel, or their alloys are exposed to heat, causing the crystal structure to transform from ferrite to austenite. This phase transformation occurs between 912 to 1,394 degrees Celsius, in which alpha iron undergoes the shift to gamma iron (austenite), which is soft and ductile and can dissolve more carbon.
The rate at which the austenite cools down and solidifies plays a critical role in determining its final state. If the cooling is done slowly, it allows carbon to diffuse out of austenite, forming ferrite and cementite or pearlite. On the other hand, rapid cooling or quenching leads to martensitic transformation, resulting in a much harder and brittle steel component.
Interestingly, alloying elements such as nickel and manganese can stabilize austenite at lower temperatures. This property is the backbone of austenitic stainless steel that remains austenitic even at room temperature.
In heat-treating processes to gauge temperature changes, the color of emitted light from cherry-red to orange-red corresponds to the austenite formation. Knowing when this formation occurs ensures that the austenite’s properties are brought out in optimal conditions, which is essential for manufacturers creating high-performance steels.
Retained Austenite
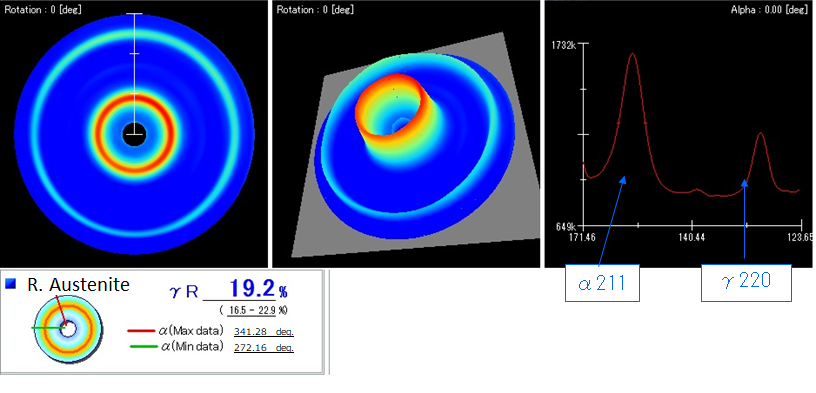
Retained austenite refers to the austenite that remains in steel after it’s been heat treated. This happens because not all of the austenite transforms into martensite during the quenching process.
It’s important to understand that retained austenite is not inherently good or bad—its ideal value largely depends on its surrounding context. The amount of allowable retained austenite will vary based on the part’s application.
For instance, some engineers intentionally manipulate heat treatment processes to control the amount of retained austenite in a steel component, balancing it with other microstructures, such as martensite, to achieve desired mechanical properties like hardness, ductility, and wear resistance. Read this article to learn more about retained austenite.
Measuring Austenite
Like martensite, one of the most common ways to measure austenite in steel is by XRD. After taking measurements, you’ll analyze the X-ray diffraction patterns and compare the intensities of specific peaks. Again, specific peaks in the XRD spectrum correspond to austenite and martensite. By comparing the intensities of these peaks, you can quantify the relative amounts of each phase. The ratio of the intensities of austenite to martensite peaks provides the percentage of retained austenite in the steel.
Measure Martensite & Austenite With Pulstec’s XRD Analyzer
Measuring austenite and martensite can be carried out using a variety of techniques, but X-ray diffraction is one of the most effective methods because it’s fast and can be performed without damaging the specimen.
You can use Pulstec’s μ-X360s portable X-ray analyzer to perform reliable measurements of steel specimens. Our analyzer is capable of displaying the full Debye-Scherrer ring. The intensity and position of the diffraction peaks in this ring can provide valuable insights into the crystal structure of the material, including levels of martensite and austenite. It can measure residual stress in as little as 60 seconds and retained austenite in 90 seconds.
Please contact us today to learn more about our X-ray analyzer, or visit our blog for more retained austenite resources.